By Leoma Bere A new and improved method of cell therapy has shown promising early…
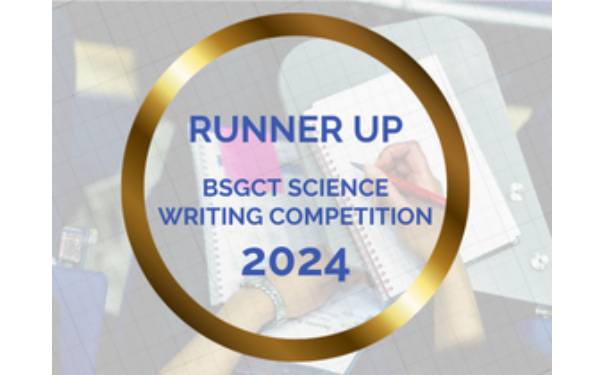
How close is an affordable CAR-T cell therapy?
By Syd McLean
This is not an easy question. Decreasing the cost of CAR-T is a complicated area of study, and the required solutions will come from both scientists, engineers, clinicians, and economists.
What is CAR-T therapy?
Chimeric Antigen Receptor T cell therapy (CAR-T) is an effective and sometimes curative treatment for aggressive cancers with limited other therapeutic options. Since the early success stories such as Emily Whitehead, the first paediatric recipient, over 25,000 patients have received CAR-T (1, 2).
In CAR-T, a receptor for a tumour cell marker is artificially added to T cells. Once the modified cells encounter this tumour in the body, the T cell killing process is triggered as shown below.
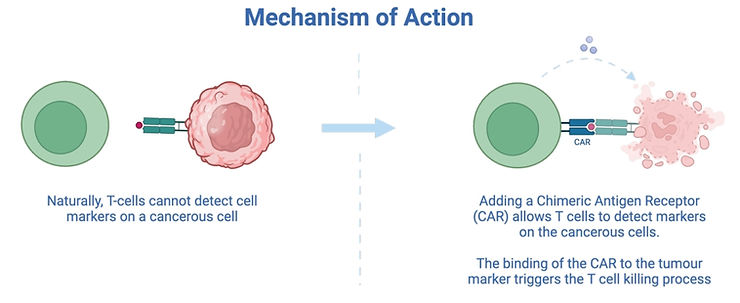
Although CAR-T is effective, it costs a lot of money. The sales cost is reported to be up to £400,000 per patient, although the exact value to health providers is often privately negotiated (3).
To answer if CAR-T can be made affordable, the first question is: what is affordable?
In the UK, some guidance is provided by the government; around £25,000 is allowed to be spent on a medicine, per quality life year it delivers to a patient (4). This guidance helps the NHS decide how to ethically spend the limited health service budget.
It is hard to estimate the exact cost effectiveness of CAR-T, but one study estimates it could be as high as £100,000 per quality life year (5). It is clear that to increase accessibility of these cutting-edge therapies to patients, costs need to be reduced.
How is CAR-T currently made?
Making a CAR-T therapy can take up to 14 days, and the method of making them varies between providers (6). For most currently approved therapies, the standard process consists of: collecting T-cells, inserting the CAR gene, growing the CAR-T cells, packaging them, and infusing to a patient.
1. Collecting T-cells
In this step white blood cells are collected from a patient and purified to extract a pure T-cell population.
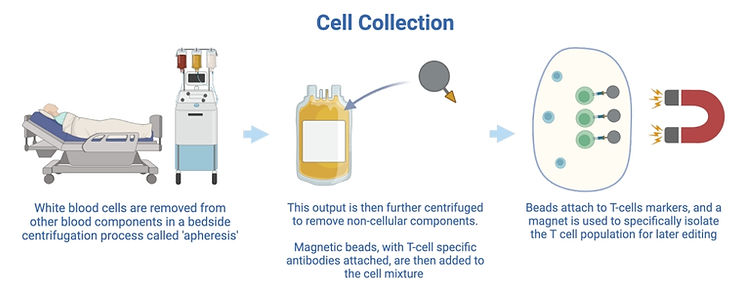
2. Inserting the CAR gene
T-cells then are genetically modified to express the Chimeric Antigen Receptor (CAR) on their cell surface. Specially engineered non-pathogenic lentiviruses are commonly used to do this. After infecting T cells, they permanently integrate the CAR gene into the host cell’s genome.

3. Growing the cells
The number of T cells extracted from a patient is usually low. For the CAR-T cells to be effective in killing tumours, the number of cells need to be increased before patient infusion. After this, a quality control step checks the quality and tumour killing ability of the CAR-T cells, and ensures no presence of pathogenic virus.
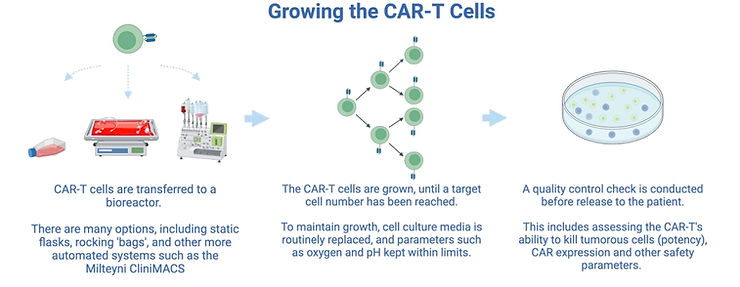
4. Packaging and administering cells
In this step the CAR-T cells are packaged into bags and frozen, for shipping to the clinic. Although this sounds trivial, it is a complicated step. This is because a cryopreservant is commonly used which is necessary to protect cells during freezing, but toxic to them at room temperature (7). Once this cryopreservant is added to the cells, there is a time pressure to freeze the bags, which must be done at a controlled rate of -1°C per minute (8). Machines to automate this step at larger scales have been identified as a priority for development (9).
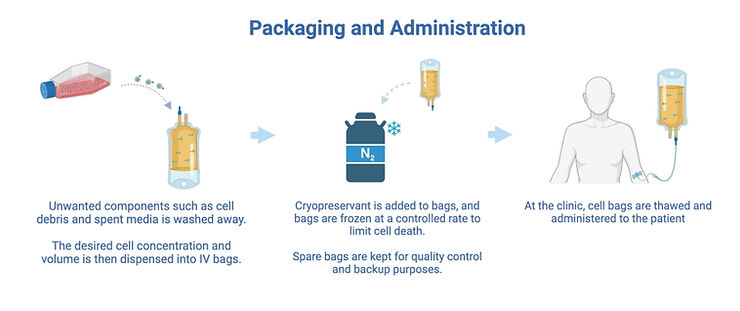
What is being done to make CAR-T cheaper?
One approach is to optimise the current manufacturing process. By measuring and controlling parameters such as oxygen, pH and glucose, target cell numbers in the growth stage can be achieved significantly faster (10). This can also reduce T cell ‘exhaustion’, shown to improve the tumour killing response in the body (11).
Different manufacturing strategies may also help, informed by research into the cost drivers of CAR-T (12). Some companies are looking to reduce labour costs by developing new instruments to automate most of the manufacturing process, such as OriBiotech, Cellares and Lonza. Another approach is to reduce instrument capital costs by creating ‘modular’ cleanroom layouts, with separate machines for each step. This may enable higher instrument utilisation, as a large proportion of manufacturing time is spent in bioreactors during the cell growth stage (13). Logistical costs may be reduced, by manufacturing at the point of care rather than a centralised factory (14). Finally, another significant cost is the raw materials, especially lentiviruses. This may be reduced with the use of non-viral gene editing methods such as mRNA, transposons and CRISPR/Cas9 (15).
There are also fundamentally different biological approaches which may reduce costs. In most currently approved therapies, the starting cells come from the sick patient. However, it is possible to instead source the cells from healthy patients, theoretically enabling economies of scale by allowing CAR-T cells to be pre-prepared in large batches. There are however some biological challenges with doing this, and this is an area of current research (16). Another radically different way of making CAR-T cells is to do the genetic editing inside the body, thereby eliminating most of the manufacturing process. Lipid nanoparticle mRNA technology, developed during the COVID-19 pandemic, may be instrumental in this approach (17).
Developments in regulations and new economic strategies may also be required to make CAR-T cheaper. Developing a CAR-T therapy and running clinical trials is extremely expensive, becoming a barrier to commercialisation when the target patient population is small (18). Regulations allowing hospitals to manufacture cell therapies themselves may help make developing CAR-T more economically feasible for treating rarer cancers (19). There is also work being done to reducing the cost needed to develop cell therapies, by making better small-scale models of the manufacturing process (20). An Indian company, ImmunoACT, has reportedly developed a significantly cheaper CAR-T therapy by making significant reductions in the labour costs involved in research and development (21).
In conclusion, there is not one ‘silver bullet’ to make CAR-T more affordable, with each strategy having its advantages and challenges. However, with the number of different approaches being developed regularly, it is certainly a promising area of research to keep an eye on.
References
1. Our Journey | Emily Whitehead Foundation [Internet]. [cited 2024 May 6]. Available from: https://emilywhiteheadfoundation.org/our-journey/.
2. Patient Access Analytics | Ori Biotech [Internet]. [cited 2024 May 6]. Available from: https://oribiotech.com/patient-access-analytics/.
3. Hwang WY, Takahashi S, Choi B, Huang H, Kawamata S, Ng SC, et al. Challenges in Global Access to CAR-T cells: an Asian Perspective. Blood Cell Therapy. Asia-Pacific Blood and Marrow Transplantation Group; 2024; 7(1):10.
4. Claxton K, Martin S, Soares M, Rice N, Spackman E, Hinde S, et al. Methods for the estimation of the National Institute for Health and Care Excellence cost-effectiveness threshold. Health Technol Assess. Health Technol Assess; 2015; 19(14):1–503.
5. Choe JH, Abdel-Azim H, Padula W V., Abou-El-Enein M. Cost-effectiveness of Axicabtagene Ciloleucel and Tisagenlecleucel as Second-line or Later Therapy in Relapsed or Refractory Diffuse Large B-Cell Lymphoma. JAMA Netw Open. American Medical Association; 2022; 5(12):e2245956–e2245956.
6. Tumaini B, Lee DW, Lin T, Castiello L, Stroncek DF, Mackall C, et al. Simplified process for the production of anti-CD19-CAR engineered T cells. Cytotherapy. NIH Public Access; 2013; 15(11):1406.
7. Haastrup EK, Munthe-Fog L, Ballesteros OR, Fischer-Nielsen A, Svalgaard JD. DMSO (Me2SO) concentrations of 1-2% in combination with pentaisomaltose are effective for cryopreservation of T cells. Transfus Apher Sci. Transfus Apher Sci; 2021; 60(4).
8. LI RUI, JOHNSON R, YU G, MCKENNA DH, HUBEL A. Preservation of cell-based immunotherapies for clinical trials. Cytotherapy. NIH Public Access; 2019; 21(9):943.
9. Dias J, Garcia J, Agliardi G, Roddie C. CAR-T cell manufacturing landscape—Lessons from the past decade and considerations for early clinical development. Mol Ther Methods Clin Dev. 2024; 32(2):101250.
10. Hood T, Slingsby F, Sandner V, Geis W, Schmidberger T, Bevan N, et al. A quality-by-design approach to improve process understanding and optimise the production and quality of CAR-T cells in automated stirred-tank bioreactors. Front Immunol. Frontiers Media SA; 2024; 15:1335932.
11. Arcangeli S, Bove C, Mezzanotte C, Camisa B, Falcone L, Manfredi F, et al. CAR T cell manufacturing from naive/stem memory T lymphocytes enhances antitumor responses while curtailing cytokine release syndrome. J Clin Invest. American Society for Clinical Investigation; 2022; 132(12).
12. Lopes AG, Noel R, Sinclair A. Cost analysis of vein-to-vein CAR T-cell therapy: automated manufacturing and supply chain. Cell Gene Ther Insights. BioInsights Publishing, Ltd.; 2020; 6(3):487–510.
13. Abou-El-Enein M, Elsallab M, Feldman SA, Fesnak AD, Heslop HE, Marks P, et al. Scalable Manufacturing of CAR T Cells for Cancer Immunotherapy. Blood Cancer Discov. American Association for Cancer Research; 2021; 2(5):408.
14. Orentas RJ, Dropulić B, Lima M de. Place of care manufacturing of chimeric antigen receptor cells: Opportunities and challenges. Semin Hematol. W.B. Saunders; 2023; 60(1):20–4.
15. Moretti A, Ponzo M, Nicolette CA, Tcherepanova IY, Biondi A, Magnani CF. The Past, Present, and Future of Non-Viral CAR T Cells. Front Immunol. Frontiers Media S.A.; 2022; 13:867013.
16. Lonez C, Breman E. Allogeneic CAR-T Therapy Technologies: Has the Promise Been Met? Cells. Multidisciplinary Digital Publishing Institute (MDPI); 2024; 13(2).
17. Billingsley MM, Gong N, Mukalel AJ, Thatte AS, El-Mayta R, Patel SK, et al. In Vivo mRNA CAR T Cell Engineering via Targeted Ionizable Lipid Nanoparticles with Extrahepatic Tropism. Small. Small; 2024; 20(11).
18. Sabatini MT, Chalmers M. The Cost of Biotech Innovation: Exploring Research and Development Costs of Cell and Gene Therapies. Pharmaceut Med [Internet]. Pharmaceut Med; 2023 [cited 2024 May 7]; 37(5):365–75. Available from: https://pubmed.ncbi.nlm.nih.gov/37286928/.
19. Becerra A, Kuhn A, Kurtoglu M. Advanced therapies, hospital exemptions & marketing authorizations: the UK’s emerging regulatory framework for point-of-care manufacture. Cell Gene Ther Insights. BioInsights Publishing, Ltd.; 2023; 09(03):315–25.
20. Costariol E, Rotondi MC, Amini A, Hewitt CJ, Nienow AW, Heathman TRJ, et al. Demonstrating the Manufacture of Human CAR-T Cells in an Automated Stirred-Tank Bioreactor. Biotechnol J. John Wiley & Sons, Ltd; 2020; 15(9):2000177.
21. Mallapaty S. Cutting-edge CAR-T cancer therapy is now made in India – at one-tenth the cost. Nature. 2024; 627(8005):709–10