Author: Saif Malik, University College London
All the blood in the human body passes through the kidney 40 times a day for the removal of waste, excess fluid, and maintenance of electrolytes(1). On its way to the kidney from the heart, the blood travels down highway aorta, splitting at numerous junctions into different organs before it reaches the renal artery, a one-way street into the kidney. Once in the kidney the blood disperses further into numerous side roads known as arterioles that terminate at small balls of tiny blood vessels surrounded by cellular capsules, together known as the glomeruli (Figure 1). The glomerulus is where blood filtration occurs, it is in
essence, the human sieve. Small waste products are allowed to leave the blood and enter the urine whereas large important molecules such as proteins are maintained(2).

If the glomerulus is damaged or dysfunctional, if your sieve is broken, large proteins such as albumin can enter the urine(3), like pasta falling into the sink (Figure 2). This is known as ‘proteinuria’ and is often the first sign of glomerular disease, a precursor for chronic kidney disease (CKD) which is estimated to affect 1 in 10 people globally(4). CKD can be caused by multiple factors but in children, mutations in the genetic code of glomerular cells, particularly the cells that form the ‘slits’ of the sieve known as podocytes, are the leading cause of glomerular disease(5). Over 50% of these children do not respond to first line treatments such as steroids and progress to end stage kidney disease between the ages of 5 and 8 at which point their only option for survival is lifelong dialysis or transplant(6). With transplant lists growing, it is of upmost importance to target CKD early before it progresses end stage kidney disease, and due to their early role in the progression of CKD, mutated glomerular podocytes are of high interest as a therapeutic target for delivery of nucleic acids such as mRNA, the building blocks for functional proteins.

But how can we target one cell type, nestled in the outer layer of the kidney with minimal off target effects? This is the question I aim to answer in my PhD.
I do this by harnessing technology similar to some of the COVID-19 vaccines distributed to millions of people across the world(7), by using small balls of fat known as lipid nanoparticles that are capable of packaging and protecting genetic cargo from the immune system(8). In order to enhance cell targeting and uptake, the nanoparticles are coated in tiny proteins called peptides that specifically bind to a receptor only found on podocytes in the kidney, in theory a lock and key for cell entry (Figure 3).
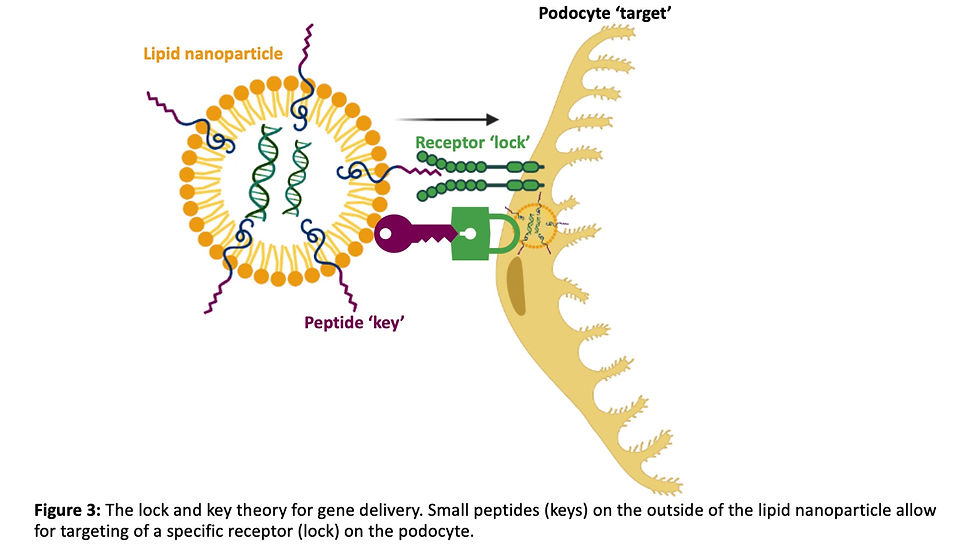
In the first part of my PhD, I found a peptide (peptide A) which is more specific for the target receptor than previously published receptor binding peptides (B and C). I deduced this by testing the ability of different peptide coated nanoparticles carrying luciferase mRNA, the protein responsible for making fireflies glow to be taken up by podocytes isolated directly from healthy mice (Figure 4A) and mice with a genetic glomerular disease (Figure 4B).
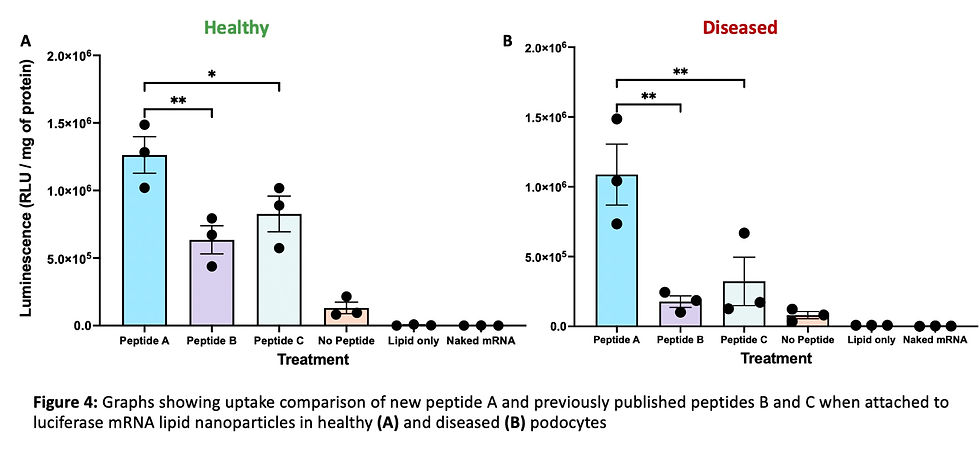
This lock and key method for gene delivery is great if you’re outside the door, but to enter the podocytes, the nanoparticle has to first be in the kidney. This is highly unlikely if we utilise the most common routes of administration such as intravenous injection, because after passing all through the body, under 5% of therapies reach the kidney due to major liver and pulmonary accumulation(9). To have any therapeutic benefit, high doses are required and therefore the chance of adverse events increases greatly.
To combat this, our group has developed a minimally invasive ultrasound guided injection through the skin directly into the renal artery(9), the one-way street into the kidney glomerulus and thus podocytes (Figure 5A). Through the use of this novel method, human derived stems cells expressing luciferase were delivered to healthy mouse kidneys which was confirmed by a strong signal via an imaging technique that allows visualisation of luciferase activity known as bioluminescent imaging (BLI). I have applied this technique to deliver luciferase mRNA carrying lipid nanoparticles to the mouse kidney and have shown that on average over 80% of the whole-body luminescent signal is accounted for by the injected kidney with negligible uptake in other organs including the liver and lungs (Figure 5B). It is evident that when compared to the 5% kidney uptake of intravenous administration, that our approach has a clear advantage at levels not observed before for delivery of genes to the kidney.

There is still however, a long way to go. Ongoing studies focus on delivering fluorescent marker genes to assess podocyte uptake following renal artery injection and we are currently analysing the best candidates to alleviate podocyte injury and therefore halt progression to end stage kidney disease in our mouse model that can hopefully be translated to the clinic.
In the metropolis of the body, it is clear that the renal artery injection allows us to bypass the aorta ‘highway’ and the side junctions that lead to different organs. Therefore, we can deliver gene therapies directly to the kidney in a minimally invasive manner at levels not possible with traditional administration routes. But most importantly, this novel approach provides us with a unique opportunity to improve the lives of children suffering from kidney disease.
References
McMahon, A. P. (2016) ‘Development of the Mammalian Kidney’, Current topics in developmental biology. 117, p. 31. doi: 10.1016/BS.CTDB.2015.10.010.
Scott, R. P. and Quaggin, S. E. (2015) ‘The cell biology of renal filtration’, Journal of Cell Biology. pp. 199–210. doi: 10.1083/jcb.201410017.
Mundel P, Shankland SJ. Podocyte biology and response to injury. J Am Soc Nephrol. 2002 Dec;13(12):3005-15. doi: 10.1097/01
Kovesdy CP. Epidemiology of chronic kidney disease: an update 2022. Kidney Int Suppl (2011). 2022 Apr;12(1):7-11. doi: 10.1016/j.kisu.2021.11.00
Li, A. S., Ingham, J. F. and Lennon, R. (2020) ‘Genetic disorders of the glomerular filtration barrier’, Clinical Journal of the American Society of Nephrology. 15(12), pp. 1818–1828.
Roca, N. et al. (2019) ‘Long-term outcome in a case series of Denys–Drash syndrome’, Clinical Kidney Journal, 12(6), pp. 836–839. doi: 10.1093/ckj/sfz022.
Baden, L. R. et al. (2020) ‘Efficacy and Safety of the mRNA-1273 SARS-CoV-2 Vaccine’, NEJM, 384(5), pp. 403–416.
Liu, C. et al. (2020) ‘Barriers and Strategies of Cationic Liposomes for Cancer Gene Therapy’, Molecular Therapy - Methods and Clinical Development, pp. 751–764. doi: 10.1016/j.omtm.2020.07.015.
Rubin, J.D., Barry, M.A. Improving Molecular Therapy in the Kidney. Mol Diagn Ther 24, 375–396 (2020)
Thin, M. Z. et al. (2019) ‘Stem cell delivery to kidney via minimally invasive ultrasound-guided renal artery injection in mice’. doi: 10.1101/609230.